Magnetic precession – the rotation of the magnetisation around an applied torque — seems an unavoidable obstacle to faster magnetic devices. Now, researchers at the LPS have shown that magnetic precession can be suppressed in certain magnetic alloys to drastically speed up the change of their magnetisation.
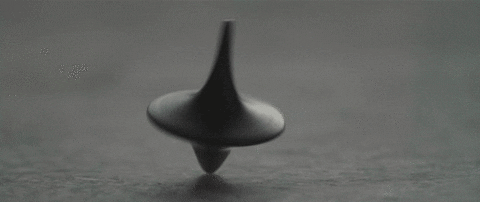
Magnetisation is a phenomenon tightly associated to rotation, arising in materials where the electrons in the atoms have a total orbital momentum or spin. This gives magnetic materials an angular momentum, just like a rotating top or any other rotating object. When a torque is applied to an object with angular momentum, like a magnetic field to switch a magnet, or the weight on a top, the rotation axis of the object circles (or “precesses”) around its new equilibrium direction for a long time before eventually reaching it. This phenomenon can be very useful: it keeps a spinning top upright, makes bicycles easier to steer, and is used in devices called gyroscopes to keep a steady referential in satellites and airplanes (see figure 1). But precession can also be detrimental. Writing information to a hard disk drive or a MRAM chip involves changing the direction of the magnetisation of small regions in a magnetic film, a process that is slowed down by magnetic precession.
In this study, researchers from the LPS have demonstrated experimentally that it is possible to suppress the precession of magnetic materials with vanishing net angular momentum, allowing for a fast and efficient manipulation of the magnetisation. The researchers studied a thin film of GdFeCo, a magnetic alloy composed of gadolinium (Gd) and iron with a bit of cobalt (Fe85Co15). In this alloy, the moments of Gd and of FeCo are anti-aligned: when the magnetisation of Gd is pointing up, the magnetisation of FeCo is pointing down (Figure 2). The angular momenta of Gd and of FeCo are opposite, and the net angular momentum is the difference between the two. If they are perfectly balanced, the net angular momentum of the alloy is zero, and the magnetic precession should be suppressed.
To detect this precessionless motion, the researchers decided to study the velocity of magnetic domain walls driven by current. A domain wall is a very small region (only a few nanometers; 1 nm = 10-9 m) that separates two domains of uniform magnetisation (Figure 2). It can be moved by applying electric currents. Crucially, the domain wall velocity depends on precession: the domain wall moves slowly when the film magnetisation precesses (about 100 m/s) and is much faster (up to km/s) when the magnetisation does not. Using an optical microscopy technique based on the modification of polarised light by the magnetisation of the film (‘Kerr Microscopy’), the position of the domain wall could be determined. The researchers then applied short electrical current pulses (25 ns long) and measured the displacement of the domain wall, and then calculated its velocity (see figure 2).
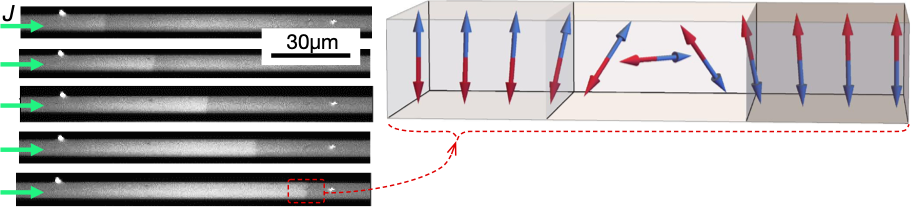
GdFeCo also has a very useful property for this study: the magnitudes of the two opposing angular momenta, of the Gd and FeCo, decrease at different rates with temperature. So, just by changing the temperature by a few degrees, it is possible to change the net momentum of the alloy, and go from a state with a net angular momentum to a state with no net momentum (at the “angular momentum compensation temperature”). This is exactly what the researchers did: they measured the velocity of the domain wall at different temperatures, and observed that it was faster at a particular temperature. To prove that this was indeed due to a change in the precession of the magnetisation of the domain wall, the researchers applied an external field along the domain wall. This field was oriented so that it rotated the magnetisation just like the precession would. By examining how the velocity changed with the applied field, the exact orientation of the magnetisation was deduced (figure 3) showing that there is no precession when the angular momenta Gd and FeCo are balanced!
With precessionless magnetisation, magnetic devices could be sped up by an order of magnitude. The switching time of MRAMs could be reduced from few ns to less than 1 ns, and domain walls velocities sped up from 100s of m/s to a few km/s, all without requiring higher currents — just what is needed for the fast and power efficient spintronic devices of tomorrow.
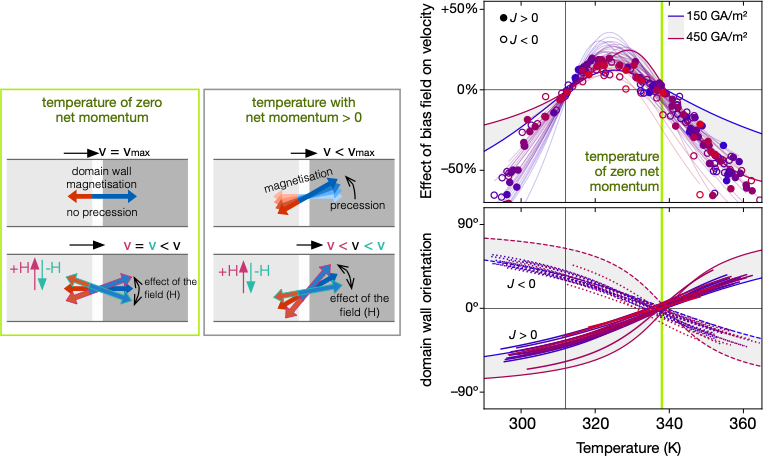
Reference
Measurement of the tilt of a moving domain wall shows precession-free dynamics in compensated ferrimagnets
E. Haltz, J. Sampaio, S. Krishnia, L. Berges, R. Weil & A. Mougin
Scientific Reports 10, 16292 (2020)
doi: 10.1038/s41598-020-73049-5