Researchers have developed a new method to introduce controlled disorder intro crystals of anisotropic gold nanoparticles. This result, at the crossroad between chemistry and engineering, offers prospects for the synthesis of materials with new functional properties.
Generally, materials produced by living organisms are neither perfectly organized (like crystals) nor completely amorphous. This in-between sweet spot is presumably at the origin of the many outstanding properties of biomaterials. When researchers try to mimic Nature in the lab using nanoparticles, simple and ordered structures are obtained because most nanoparticles are symmetrical and do not easily yield complex assemblies. To solve this issue, one needs to control the environment of the particles in order to guide their self-assembly. Forcing nanoparticles to assemble into tiny spaces, such as liquid droplets, could be used as a way to obtain intricate structures. However, this strategy has limitations: while the particle size and concentration within the droplet, as well as the total volume, are easily controlled, the droplet shape stays round. This is a major drawback, as curved interfaces are typically less effective in aligning the nanoparticles in the final material.
By using flat and hard surfaces instead of curved ones, scientists have been able to guide particles more precisely. A long-standing collaboration involving the LPS (CNRS/University of Paris-Saclay), the ICS (CNRS/University of Strasbourg) and the SWING beamline of the SOLEIL synchrotron demonstrated the self-assembly of nanoparticles inside tiny cavities of several different shapes. The interfaces of these molds guide the growth of multiple crystal domains, allowing fine control over the orientation of nanoparticles and the formation of defects in the final materials (Figure). This work has been published in the journal ACS Nano.
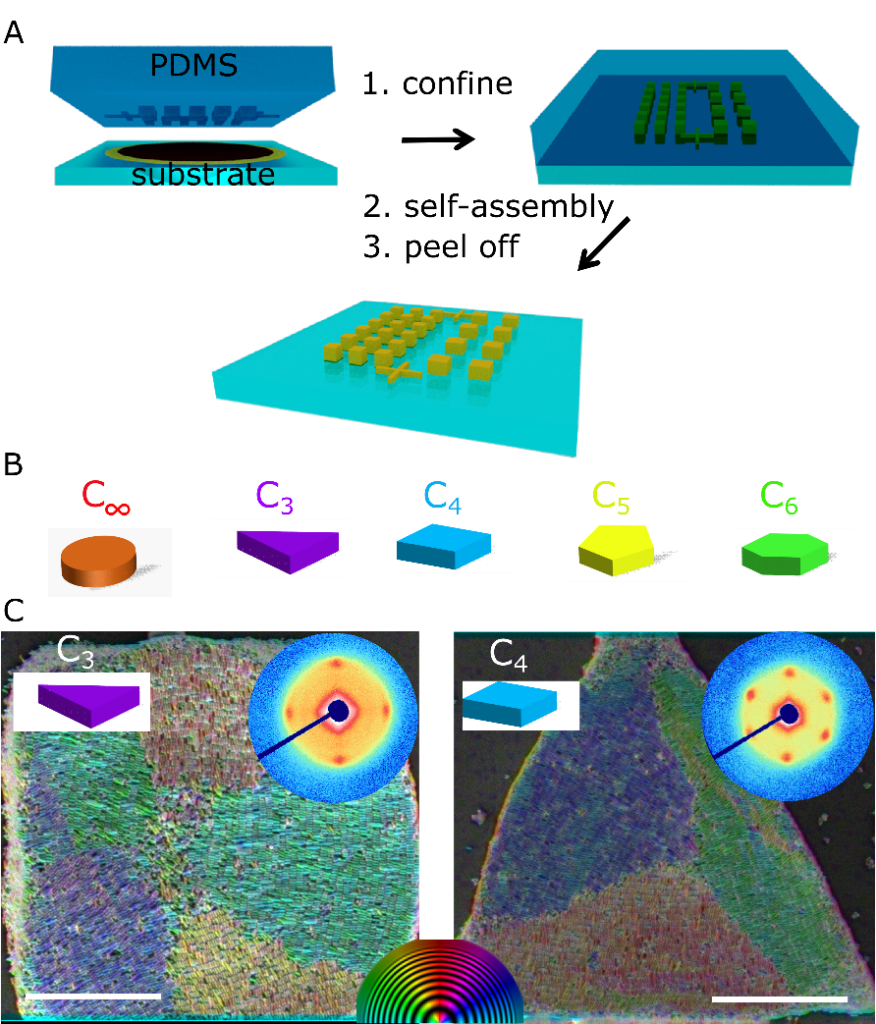
Figure. Principle of the method. A) Schematic view of the templated evaporation-induced self-assembly process. B) Schematic pictures of the investigated microcavity shapes. C) scanning electron microscopy images of the self-assembled nanorods in cavities shaped like a triangular prism and a square prism. The nanorods are color-encoded according to their orientation, in order to distinguish the different crystalline domains. Scale bar is 1 µm on both SEM images. The insets are the corresponding two-dimensional SAXS images, which confirm the alignment of nanorods across the much larger collection of cavities (300 × 300 µm2).
Gold nanoparticles with varying shapes were assembled inside prism-shaped cavities by evaporation from solution. By studying these structures at different scales, we learned how to control their orientation by changing the shape of the confining cavities. We analyzed the orientation of these supercrystals over large scales using conventional small angle X-ray scattering (SAXS), but also for each individual assembly using microbeam SAXS (µSAXS), while scanning electron microscopy (SEM) helps us understand their real-space structure. Our findings show that different crystal patterns formed depending on the shape of the cavity, with each side of the cavity directing the growth of one crystal domain until it met another, forming grain boundaries between them. Computer simulations showed that similar patterns appeared when the nanoparticles were squeezed into cavities with polygonal cross section. This method worked both for gold nanorods and bipyramids, suggesting it could be applied to a wide variety of nanoparticles.
This research could have applications in fields like plasmonics, where structured materials can interact with light in specific ways. For example, by controlling the orientation of these structures, we could make surfaces that are sensitive to different polarizations of light. Beyond plasmonics, this method could be used to control the emission of light from semiconducting materials. Overall, this work holds significance from fundamental interest to the development of unconventional optical elements.
Reference
Prismatic Confinement Induces Tunable Orientation in Plasmonic Supercrystals
W. Chaâbani, J. Lyu, J. Marcone, C. Goldmann, E. J. M. ten Veen, C. Dumesnil, T. Bizien, F. Smallenburg, M. Impéror-Clerc, D. Constantin, C. Hamon
ACS Nano 18, 9566-9575 (2024)
DOI: 10.1021/acsnano.3c12799
Open archive platforms: HAL